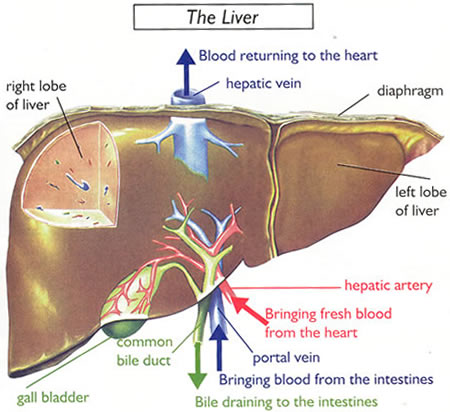
Hepatic encephalopathy – awesome article
Hepatic encephalopathy – awesome article
Author: David C Wolf, MD, FACP, FACG, AGAF, Medical Director of Liver Transplantation, Westchester Medical Center, Professor of Clinical Medicine, Division of Gastroenterology and Hepatobiliary Diseases, Department of Medicine, New York Medical College
Contributor Information and Disclosures
Updated: Mar 25, 2010
Definition
Hepatic encephalopathy is a syndrome observed in patients with cirrhosis. Hepatic encephalopathy is defined as a spectrum of neuropsychiatric abnormalities in patients with liver dysfunction, after exclusion of other known brain disease. Hepatic encephalopathy is characterized by personality changes, intellectual impairment, and a depressed level of consciousness.
An important prerequisite for the syndrome is diversion of portal blood into the systemic circulation through portosystemic collateral vessels. Hepatic encephalopathy is also described in patients without cirrhosis with either spontaneous or surgically created portosystemic shunts.
The development of hepatic encephalopathy is explained, to some extent, by the effect of neurotoxic substances, which occurs in the setting of cirrhosis and portal hypertension.
Subtle signs of hepatic encephalopathy are observed in nearly 70% of patients with cirrhosis. Symptoms may be debilitating in a significant number of patients and are observed in 24-53% of patients who undergo portosystemic shunt surgery. Approximately 30% of patients dying of end-stage liver disease experience significant encephalopathy, approaching coma.
Hepatic encephalopathy, accompanying the acute onset of severe hepatic synthetic dysfunction, is the hallmark of fulminant hepatic failure (FHF). Symptoms of encephalopathy in FHF are graded using the same scale used to assess encephalopathy symptoms in cirrhosis.
The encephalopathy of cirrhosis and FHF share many of the same pathogenic mechanisms. However, brain edema plays a much more prominent role in FHF than in cirrhosis. The brain edema of FHF is attributed to increased permeability of the blood-brain barrier, impaired osmoregulation within the brain, and increased cerebral blood flow.
The resulting brain cell swelling and brain edema are potentially fatal. In contrast, brain edema is rarely reported in patients with cirrhosis. The encephalopathy of FHF is not covered in this article but is addressed in Acute Liver Failure.
A nomenclature has been proposed for categorizing hepatic encephalopathy. Type A hepatic encephalopathy describes encephalopathy associated with A cute liver failure. Type B hepatic encephalopathy describes encephalopathy associated with portal-systemic Bypass and no intrinsic hepatocellular disease. Type C hepatic encephalopathy describes encephalopathy associated with Cirrhosis and portal hypertension or portal-systemic shunts. Type C hepatic encephalopathy is, in turn, subcategorized as episodic, persistent, or minimal.
For excellent patient education resources, visit eMedicine’s Liver, Gallbladder, and Pancreas Center and Hepatitis Center. Also, see eMedicine’s patient education article Cirrhosis.
Pathogenesis
A number of theories have been proposed to explain the development of hepatic encephalopathy in patients with cirrhosis. Some investigators contend that hepatic encephalopathy is a disorder of astrocyte function. Astrocytes account for about one third of cortical volume. They play a key role in the regulation of the blood-brain barrier. They are involved in maintaining electrolyte homeostasis and in providing nutrients and neurotransmitter precursors to neurons. They also play a role in the detoxification of a number of chemicals, including ammonia.4
It is theorized that neurotoxic substances, including ammonia and manganese, may gain entry into the brain in the setting of liver failure. These neurotoxic substances may then contribute to morphologic changes in astrocytes. In cirrhosis, astrocytes may undergo Alzheimer type II astrocytosis. Here, astrocytes become swollen. They may develop a large pale nucleus, a prominent nucleolus, and margination of chromatin.
In FHF, astrocytes may also become swollen. The changes of Alzheimer type II astrocytosis are not seen in FHF. But, in contrast to cirrhosis, astrocyte swelling in FHF may be so marked as to produce brain edema. This may lead to increased intracranial pressure and, potentially, brain herniation.
In the late 1990s, authors from the University of Nebraska, using epidural catheters to measure intracranial pressure (ICP), reported elevated ICP in 12 patients with advanced cirrhosis and grade 4 hepatic coma over a 6-year period.5 Cerebral edema was reported on CT scan of the brain in 9 of the 12 patients.
Several of the patients transiently responded to treatments that are typically associated with the management of cerebral edema in patients with FHF. Interventions included elevation of the head of the bed, hyperventilation, intravenous mannitol, and phenobarbital-induced coma.
In the author’s opinion, patients with worsening encephalopathy should undergo head CT scan to rule out the possibility of an intracranial lesion, including hemorrhage. Certainly, cerebral edema, if discovered, should be aggressively managed. The true incidence of elevated ICP in patients with cirrhosis and severe hepatic encephalopathy remains to be determined.
Work focused on changes in gene expression in the brain has been conducted. The genes coding for a wide array of transport proteins may be upregulated or downregulated in cirrhosis and FHF. As an example, the gene coding for the peripheral-type benzodiazepine receptor is upregulated in both cirrhosis and FHF. Such alterations in gene expression may ultimately result in impaired neurotransmission.
Hepatic encephalopathy may also be thought of as a disorder that is the end result of accumulated neurotoxic substances in the brain. Putative neurotoxins include short-chain fatty acids; mercaptans; false neurotransmitters, such as tyramine, octopamine, and beta-phenylethanolamines; manganese; ammonia; and gamma-aminobutyric acid (GABA).
Ammonia hypothesis
Ammonia is produced in the gastrointestinal tract by bacterial degradation of amines, amino acids, purines, and urea. Enterocytes also convert glutamine to glutamate and ammonia by the activity of glutaminase.
Normally, ammonia is detoxified in the liver by conversion to urea by the Krebs-Henseleit cycle. Ammonia is also consumed in the conversion of glutamate to glutamine, a reaction that depends upon the activity of glutamine synthetase. Two factors contribute to the hyperammonemia that is seen in cirrhosis.
First, there is a decreased mass of functioning hepatocytes, resulting in fewer opportunities for ammonia to be detoxified by the above processes. Secondly, portosystemic shunting may divert ammonia-containing blood away from the liver to the systemic circulation.
Normal skeletal muscle cells do not possess the enzymatic machinery of the urea cycle but do contain glutamine synthetase. Glutamine synthetase activity in muscle actually increases in the setting of cirrhosis and portosystemic shunting. Thus, skeletal muscle is an important site for ammonia metabolism in cirrhosis. However, the muscle wasting that is observed in patients with advanced cirrhosis may potentiate hyperammonemia.
The kidneys express glutaminase and, to some extent, play a role in ammonia production. However, the kidneys also express glutamine synthetase and play a key role in ammonia metabolism and excretion.
Brain astrocytes also possess glutamine synthetase. However, the brain is not able to increase glutamine synthetase activity in the setting of hyperammonemia. Thus, the brain remains vulnerable to the effects of hyperammonemia.
Ammonia has multiple neurotoxic effects. It can alter the transit of amino acids, water, and electrolytes across astrocytes and neurons. It can impair amino acid metabolism and energy utilization in the brain. Ammonia can also inhibit the generation of excitatory and inhibitory postsynaptic potentials.
Additional support for the ammonia hypothesis comes from the clinical observation that treatments that decrease blood ammonia levels can improve hepatic encephalopathy symptoms.
One argument against the ammonia hypothesis is the observation that approximately 10% of patients with significant encephalopathy have normal serum ammonia levels. Furthermore, many patients with cirrhosis have elevated ammonia levels without evidence for encephalopathy.
Also, ammonia does not induce the classic electroencephalographic (EEG) changes associated with hepatic encephalopathy when it is administered to patients with cirrhosis.
GABA hypothesis
GABA is a neuroinhibitory substance produced in the gastrointestinal tract. Of all brain nerve endings, 24-45% may be GABAergic. For 20 years, it was postulated that hepatic encephalopathy was the result of increased GABAergic tone in the brain.9 However, experimental work is changing perceptions regarding the activity of the GABA receptor complex in cirrhosis.6,10
The GABA receptor complex contains binding sites for GABA, benzodiazepines, and barbiturates. It was believed that there were increased levels of GABA and endogenous benzodiazepines in plasma. These chemicals would then cross an extrapermeable blood-brain barrier. Binding of GABA and benzodiazepines to a supersensitive neuronal GABA receptor complex permitted the influx of chloride ions into the postsynaptic neuron, leading to generation of an inhibitory postsynaptic potential.
However, experimental work has demonstrated that there is no change in brain GABA or benzodiazepine levels. Similarly, there is no change in sensitivity of the receptors of the GABA receptor complex.
Previously, it was believed that administration of flumazenil, a benzodiazepine receptor antagonist, could improve mental function in patients with hepatic encephalopathy. It now appears that flumazenil improves mental function in only a small percentage of patients with cirrhosis.
The neuronal GABA receptor complex contains a binding site for neurosteroids. Today, some investigators contend that neurosteroids play a key role in hepatic encephalopathy.
In experimental models, neurotoxins, like ammonia and manganese, increase the production of the peripheral-type benzodiazepine receptor (PTBR) in astrocytes.11 PTBR, in turn, stimulates the conversion of cholesterol to pregnenolone to neurosteroids. Neurosteroids are then released from the astrocyte. They are capable of binding to their receptor within the neuronal GABA receptor complex and can increase inhibitory neurotransmission.
One study compared the levels of various chemicals in autopsied brain tissue from patients with cirrhosis who either died in hepatic coma or died without evidence of hepatic encephalopathy. Elevated levels of allopregnanolone, the neuroactive metabolite of pregnenolone, were found in the brain tissue of patients who died in hepatic coma.12 Brain levels of benzodiazepine receptor ligands were not significantly elevated in patients with or without coma. This work further bolsters the role of neurosteroids in hepatic encephalopathy.
Clinical Features of Hepatic Encephalopathy
Grading of the symptoms of hepatic encephalopathy is performed according to the so-called West Haven classification system:13
Grade 0 – Minimal hepatic encephalopathy (previously known as subclinical hepatic encephalopathy). Lack of detectable changes in personality or behavior. Minimal changes in memory, concentration, intellectual function, and coordination. Asterixis is absent.
Grade 1 – Trivial lack of awareness. Shortened attention span. Impaired addition or subtraction. Hypersomnia, insomnia, or inversion of sleep pattern. Euphoria, depression, or irritability. Mild confusion. Slowing of ability to perform mental tasks. Asterixis can be detected.
Grade 2 – Lethargy or apathy. Disorientation. Inappropriate behavior. Slurred speech. Obvious asterixis. Drowsiness, lethargy, gross deficits in ability to perform mental tasks, obvious personality changes, inappropriate behavior, and intermittent disorientation, usually regarding time.
Grade 3 – Somnolent but can be aroused, unable to perform mental tasks, disorientation about time and place, marked confusion, amnesia, occasional fits of rage, present but incomprehensible speech
Grade 4 – Coma with or without response to painful stimuli
In minimal hepatic encephalopathy, patients may have normal abilities in the areas of memory, language, construction, and pure motor skills. However, patients with minimal hepatic encephalopathy demonstrate impaired complex and sustained attention. They may have delays in choice reaction time. They may even have impaired fitness to drive.Typically, patients with minimal hepatic encephalopathy have normal function on standard mental status testing but abnormal psychometric testing. Neurophysiologic tests in common use are the number connection test, the digit symbol test, the block design test, and tests of reaction times to light or sound (eg, critical flicker test)
Patients with mild and moderate hepatic encephalopathy demonstrate decreased short-term memory and concentration upon mental status testing. They may show signs of asterixis, although the flapping tremor of the extremities is also observed in patients with uremia, pulmonary insufficiency, and barbiturate toxicity.
Some patients show evidence of fetor hepaticus, a sweet musty aroma of the breath that is believed to be secondary to the exhalation of mercaptans.
Other potential physical examination findings include hyperventilation and decreased body temperature.
Laboratory Abnormalities in Hepatic Encephalopathy
An elevated blood ammonia level is the classic laboratory abnormality reported in patients with hepatic encephalopathy.8 This finding may aid in correctly diagnosing patients with cirrhosis who present with altered mental status. However, serial ammonia measurements are inferior to clinical assessment in gauging improvement or deterioration in a patient under therapy for hepatic encephalopathy.
Checking the ammonia level in a patient with cirrhosis who does not have hepatic encephalopathy has no utility. Only arterial or free venous blood specimens must be assayed when checking the ammonia level. Blood drawn from an extremity to which a tourniquet has been applied may provide a falsely elevated ammonia level when analyzed.
Classic EEG changes associated with hepatic encephalopathy are high-amplitude low-frequency waves and triphasic waves. However, these findings are not specific for hepatic encephalopathy. When seizure activity must be ruled out, an EEG may be helpful in the initial workup of a patient with cirrhosis and altered mental status.
Visual evoked responses also demonstrate classic patterns associated with hepatic encephalopathy. However, this test is not in common clinical use.
Computed tomography (CT) and magnetic resonance imaging (MRI) studies of the brain may be important in ruling out intracranial lesions when the diagnosis of hepatic encephalopathy is in question. MRI has the additional advantage of being able to demonstrate hyperintensity of the globus pallidus on T1-weighted images, a finding that is commonly described in hepatic encephalopathy. This finding may correlate with increased manganese deposition within this portion of the brain.
Common Precipitants of Hepatic Encephalopathy
Some patients with a history of hepatic encephalopathy may have normal mental status while under treatment. Others have chronic memory impairment in spite of medical management. Both groups of patients are subject to episodes of worsened encephalopathy. Common precipitating factors are as follows:
Renal failure:
Renal failure leads to decreased clearance of urea, ammonia, and other nitrogenous compounds.
Gastrointestinal bleeding:
The presence of blood in the upper gastrointestinal tract results in increased ammonia and nitrogen absorption from the gut. Bleeding may predispose to kidney hypoperfusion and impaired renal function. Blood transfusions may result in mild hemolysis, with resulting elevated blood ammonia levels.
Infection:
Infection may predispose to impaired renal function and to increased tissue catabolism, both of which increase blood ammonia levels.
Constipation:
Constipation increases intestinal production and absorption of ammonia.
Medications: Drugs that act upon the central nervous system, such as opiates, benzodiazepines, antidepressants, and antipsychotic agents, may worsen hepatic encephalopathy.
Diuretic therapy:
Decreased serum potassium levels and alkalosis may facilitate the conversion of NH4 + to NH3.
Dietary protein overload:
This is an infrequent cause of hepatic encephalopathy.
Differential Diagnosis for Hepatic Encephalopathy
Distinguishing hepatic encephalopathy from other acute and chronic causes of altered mental status may be difficult in patients with cirrhosis. A decision to perform additional neurologic studies should be based on the severity of the patient’s mental dysfunction, the presence of focal neurologic findings (observed infrequently in patients with hepatic encephalopathy), and the patient’s responsiveness to an empiric trial with cathartic agents. Even patients with severe hepatic encephalopathy should demonstrate steady improvement in mental dysfunction after an initiation of treatment with lactulose or cathartics derived from polyethylene glycol (PEG).
Sharma et al studied whether lactulose prevented recurrence of hepatic encephalopathy. Patients with cirrhosis who were recovering from hepatic encephalopathy were randomized to receive lactulose (n = 61) or placebo (n = 64). Over a median follow-up of 14 months, 12 patients (19.6%) in the lactulose group developed hepatic encephalopathy as compared with 30 patients (46.8%) in the placebo group (P = 0.001). The authors concluded that use of lactulose effectively prevents the recurrence of hepatic encephalopathy in patients with cirrhosis.
Differential diagnoses of encephalopathy
Intracranial lesions, such as subdural hematoma, intracranial bleeding, stroke, tumor, and abscess
Infections, such as meningitis, encephalitis, and intracranial abscess
Metabolic encephalopathy, such as hypoglycemia, electrolyte imbalance, anoxia, hypercarbia, and uremia
Hyperammonemia from other causes, such as secondary to ureterosigmoidostomy and inherited urea cycle disorders
Toxic encephalopathy from alcohol intake, such as acute intoxication, alcohol withdrawal, and Wernicke encephalopathy
Toxic encephalopathy from drugs, such as sedative hypnotics, antidepressants, antipsychotic agents, and salicylates
Organic brain syndrome
Postseizure encephalopathy
Management of Hepatic Encephalopathy
The approach to the patient with hepatic encephalopathy depends upon the severity of mental status changes and upon the certainty of the diagnosis. As an example, a patient with known cirrhosis and mild complaints of decreased concentration might be served best by an empiric trial of rifaximin or lactulose and a follow-up office visit to check its effect. However, the patient presenting in hepatic coma requires a different approach. General management recommendations include the following:
Exclude nonhepatic causes of altered mental function.
Consider checking an arterial ammonia level in the initial assessment of a hospitalized patient with cirrhosis and with impaired mental function. Ammonia levels have less use in a stable outpatient.
Precipitants of hepatic encephalopathy, such as metabolic disturbances, gastrointestinal bleeding, infection, and constipation, should be corrected.
Avoid medications that depress central nervous system function, especially benzodiazepines. Patients with severe agitation and hepatic encephalopathy may receive haloperidol as a sedative. Treating patients who present with coexisting alcohol withdrawal and hepatic encephalopathy is particularly challenging. These patients may require therapy with benzodiazepines in conjunction with lactulose and other medical therapies for hepatic encephalopathy.
Patients with severe encephalopathy (ie, grade 3 or 4) who are at risk for aspiration should undergo prophylactic endotracheal intubation. They are optimally managed in the intensive care unit.
Fanelli et al investigated the efficacy of using an hourglass-shaped expanded polytetrafluoroethylene (ePTFE) stent-graft to treat patients whose hepatic encephalopathy was refractory to conventional medical therapy. In the study, 12 patients who, subsequent to receiving a transjugular intrahepatic portosystemic shunt, had developed refractory hepatic encephalopathy underwent shunt reduction with the stent-graft.
The reduction procedure immediately produced a portosystemic gradient increase in the above study’s patients, who, within 18-26 hours after insertion of the stent-graft, no longer exhibited symptoms of hepatic encephalopathy. The condition did not recur over a mean follow-up period of 74 weeks. Over the course of the study, 4 patients died of cardiovascular failure, another underwent orthotopic liver transplantation, and 2 more were lost to follow-up. The 5 remaining patients finished the study in good clinical condition. The authors concluded that hourglass-shaped ePTFE stent-grafts appear to effectively reduce shunt flow and quickly improve patients’ clinical conditions.
Most current therapies are designed to treat the hyperammonemia that is a hallmark of most cases of hepatic encephalopathy.
Treatments to decrease intestinal ammonia production
Diet
In the late 19th century, it was recognized that the feeding of a high-protein to dogs that had undergone portosystemic shunt surgery could produce symptoms of abnormal coordination and stupor in the treated animals.
In the 20th century, low-protein diets were routinely recommended for patients with cirrhosis, in hopes of decreasing intestinal ammonia production and of preventing exacerbations of hepatic encephalopathy. An obvious consequence was the worsening of preexisting protein-energy malnutrition. Protein restriction may be appropriate in some patients immediately following a severe flare of symptoms (ie, episodic hepatic encephalopathy). However, protein restriction is rarely justified in patients with cirrhosis and persistent hepatic encephalopathy. Indeed, malnutrition is a more serious clinical problem than hepatic encephalopathy for many of these patients.
In the author’s experience, it is the infrequent patient who is intolerant of a diet high in protein. Most patients with mild chronic hepatic encephalopathy tolerate more than 60-80 g of protein per day. Furthermore, one study administered a protein-rich diet (>1.2 g/kg/d) to patients with advanced disease awaiting liver transplantation, without inducing a flare of encephalopathy symptoms.
Another study randomized patients with severe episodic encephalopathy to either a low-protein diet or a high-protein diet, administered via nasogastric tube. All patients received the same regimen of neomycin per nasogastric tube. Mental function improved at the same rate in both treatment groups. Importantly, patients receiving the low-protein diet had evidence for increased protein breakdown during the duration of the study.
Diets containing vegetable proteins appear to be better tolerated than diets rich in animal protein, especially proteins derived from red meats. This may be because of increased content of dietary fiber, a natural cathartic, and decreased levels of aromatic amino acids. Aromatic amino acids, as precursors for the false neurotransmitters tyramine and octopamine, are thought to inhibit dopaminergic neurotransmission and worsen hepatic encephalopathy.
The author recommends that patients consume well-cooked chicken and fish in addition to vegetable protein. Malnourished patients are encouraged to add commercially available liquid nutritional supplements to their diet. Patients infrequently require specialized treatment with oral or enteral supplements rich in branched-chain amino acids.
Cathartics
Lactulose (beta-galactosidofructose) and lactilol (beta-galactosidosorbitol) are nonabsorbable disaccharides that have been in common clinical use since the early 1970s (the latter is not available in the United States). They are degraded by intestinal bacteria to lactic acid and other organic acids.
Lactulose appears to inhibit intestinal ammonia production by a number of mechanisms. The conversion of Lactulose to lactic acid results in acidification of the gut lumen. This favors conversion of NH4 + to NH3 and the passage of NH3 from tissues into the lumen. Gut acidification inhibits ammoniagenic coliform bacteria, leading to increased levels of nonammoniagenic lactobacilli. Lactulose also works as a cathartic, reducing colonic bacterial load.
Initial lactulose dosing is 30 mL orally, daily or twice daily. The dose may be increased as tolerated. Patients should be instructed to reduce lactulose dosing in the event of diarrhea, abdominal cramping, or bloating. Patients should take sufficient lactulose as to have 2-4 loose stools per day.
Great care must be taken when prescribing lactulose. Overdosage can result in ileus, severe diarrhea, electrolyte disturbances, and hypovolemia. Hypovolemia may be sufficiently severe as to actually induce a flare of encephalopathy symptoms.
High doses of lactulose (eg, 30 mL q2-4h) may be administered orally or by nasogastric tube to patients hospitalized with severe hepatic encephalopathy. Lactulose may be administered as an enema to patients who are comatose and unable to take the medication by mouth. The recommended dosing is 300 mL lactulose plus 700 mL water, administered as a retention enema every 4 hours as needed. The author has had excellent success using PEG-containing colonic lavage solutions, such as Go-LYTELY administered via nasogastric tube, in the acute management of hospitalized patients with severe hepatic encephalopathy.
Lactulose has been the subject of dozens of clinical trials over almost 4 decades. Many small trials demonstrated the medication’s efficacy in the treatment of hepatic encephalopathy. However, one meta-analysis contradicts these trials and the author’s clinical experience.
When assessing high-quality randomized trials, lactulose was no more effective than placebo at improving encephalopathy symptoms. In trials comparing lactulose to an antibiotic (eg, neomycin, rifaximin), lactulose was actually inferior to antibiotic therapy. This meta-analysis certainly forces reconsideration of the use of antibiotics, particularly rifaximin.
Antibiotics
Neomycin and other antibiotics, such as metronidazole, oral vancomycin, paromomycin, and oral quinolones, are administered in an effort to decrease the colonic concentration of ammoniagenic bacteria. Initial neomycin dosing is 250 mg orally 2-4 times a day. Doses as high as 4000 mg/d may be administered. Neomycin is usually reserved as a second-line agent, after initiation of treatment with lactulose. Long-term treatment with this oral aminoglycoside runs the risks of inducing ototoxicity and nephrotoxicity because of some systemic absorption.
Rifaximin (Xifaxan, Salix Pharmaceuticals, Inc, Morrisville, NC), a nonabsorbable derivative of rifampin, has been used in Europe for 18 years for a wide variety of gastrointestinal indications. It has also been used in the treatment of hepatic encephalopathy.
In March 2010, rifaximin was approved by the Food and Drug Administration (FDA) to reduce recurrence of hepatic encephalopathy. The approval was based on a phase 3 clinical trial conducted by Bass et al.
Bass et al evaluated rifaximin’s ability to reduce the risk of recurrent hepatic encephalopathy (HE).26 In this double-blind, placebo-controlled, multinational, phase 3 clinical trial, 299 patients received either rifaximin 550 mg or placebo BID. Each group also received lactulose. The primary endpoint — the risk of a recurrent HE episode — was reduced by 58% in the rifaximin group compared with the placebo group (P <0.0001). The key secondary endpoint — risk of experiencing HE-related hospitalization — was reduced by 50% in patients who received rifaximin compared with those who received placebo (P = 0.0129).
In 2004, the drug received approval by the FDA in the United States for the treatment of travelers’ diarrhea. In 2005, it received orphan drug status as a treatment for hepatic encephalopathy. In contrast to neomycin, its tolerability profile is comparable to placebo. Multiple clinical trials have demonstrated that rifaximin at a dose of 400 mg taken orally 3 times a day was as effective as lactulose or lactilol at improving hepatic encephalopathy symptoms.
Similarly, rifaximin was as effective as neomycin and paromomycin. Rifaximin was better tolerated than both the cathartics and the other nonabsorbable antibiotics.
A number of concerns remain regarding rifaximin’s role in the treatment of hepatic encephalopathy. It remains to be determined if rifaximin can improve severe encephalopathy symptoms as rapidly as lactulose.
There are also concerns regarding the cost-effectiveness of the medication. At the author’s institution, one day’s treatment with lactulose (30 g PO qid) costs $2.20; one day’s treatment with rifaximin (400 mg PO tid) costs $18.42. However, the differential in cost of medication might be overcome if it was determined that rifaximin decreased the incidence of significant medication-related adverse effects (eg, severe abdominal cramping) or reduced hospital stay.
It also needs to be determined if rifaximin is effective at a lower dose (eg, 400 mg PO bid). Similarly, investigators need to study whether patients with persistent, mild encephalopathy can be given a drug “holiday” (eg, discontinue treatment for 1 week out of 4 weeks). There are also questions whether long-term treatment with rifaximin can induce microbial resistance. Thus far, microbial resistance has not been reported with the use of the medication.
Treatments to increase ammonia clearance
L-ornithine L-aspartate (LOLA)
LOLA (Hepa-Merz, Merz Pharmaceuticals GmbH, Frankfurt am Main, Germany) is available in Europe in both intravenous formulations and oral formulations. It is not available in the United States. LOLA is a stable salt of the 2 constituent amino acids. L-ornithine stimulates the urea cycle, with resulting loss of ammonia. Both l-ornithine and l-aspartate are substrates for glutamate transaminase. Their administration results increased glutamate levels. Ammonia is subsequently used in the conversion of glutamate to glutamine by glutamine synthetase. LOLA was found to be effective in treating hepatic encephalopathy in a number of European trials.
Zinc
Zinc deficiency is common in cirrhosis. Even in patients who are not zinc deficient, zinc administration has the potential to improve hyperammonemia by increasing the activity of ornithin